The implications of tipping points are not thoroughly quantified in the major Intergovernmental Panel on Climate Change (IPCC) analyses that inform policymakers, in part because scientists have not known enough about their mechanisms to accurately assess the potential impact and risk. What is known is:
- Tipping points exist in the climate system, and it is very unlikely that all tipping points are known;
- Different tipping points are understood with different levels of confidence, they operate on different timescales, can interact to trigger cascades of abrupt changes, and some tipping point changes are irreversible on timescales of centuries to millennia;
- The risk of tipping points occurring increases with global mean warming1. As we pass the 1.5°C target and move towards the 2.0°C ceiling for global warming under the Paris agreement some tipping points will be passed. Beyond 2°C of warming the risk of passing multiple tipping points will continue to rise;
- Our models lack the mechanisms to robustly simulate many tipping points, and the interactions between tipping points that could lead to cascading impacts, and therefore our understanding of the risks is limited;
- Since the risk is hard to quantify, global negotiations around climate change have not appropriately taken into account the risks of initiating tipping points, which is essentially a gamble on the future of the Earth’s climate;
- Resolving the risks of tipping points by proper incorporation of the mechanisms in state-of-the-art climate systems models is possible but requires a far more integrated and organised international effort.
Introduction
The term “tipping point” is commonly used in the media with language hinting at the inevitability of rapid and potentially irreversible changes being triggered at different global warming levels. “Tipping points” have also entered into the lexicon in the context of regional climate change, such as suggestions that the 2019/20 Australian drought and bushfires were evidence of a tipping point. What is the state of the science around tipping points, what evidence is there around how close we are to different tipping points, and can we predict them?
What are tipping points?
A tipping point refers to a critical threshold beyond which components of the Earth rapidly move to a new state. A tipping point leads to an abrupt, and often irreversible change that is much faster than the climate trend that is forcing the change. The timescales over which tipping point transitions take place vary widely. In some cases, abrupt change can initiate rapid cascading impacts where changes in one Earth system component lead to abrupt changes in other components, or when changes propagate from larger to smaller spatial scales. In other cases, a change can be committed to, but the timescale over which the change is actually realised can be a millennium.
Examples of potential future tipping points with harmful societal consequences include ice sheet collapse, permafrost thaw, slowdown of the Atlantic Meridional Ocean Circulation (including the Gulf Stream and thermohaline circulation with the deep ocean), ocean deoxygenation, ocean acidification and die-back of the Amazon rainforest (see Figure 1). Not all elements of the climate system exhibit tipping point behaviour. Arctic sea ice, for example, is likely to decline proportionally with increasing warming, and is therefore probably not subject to tipping points. Sea ice is also expected to be recoverable if climate warming is reversed, but the impacts of rapid loss of Arctic sea ice and associated amplification of warming in the Arctic may trigger other tipping points in the Earth system.
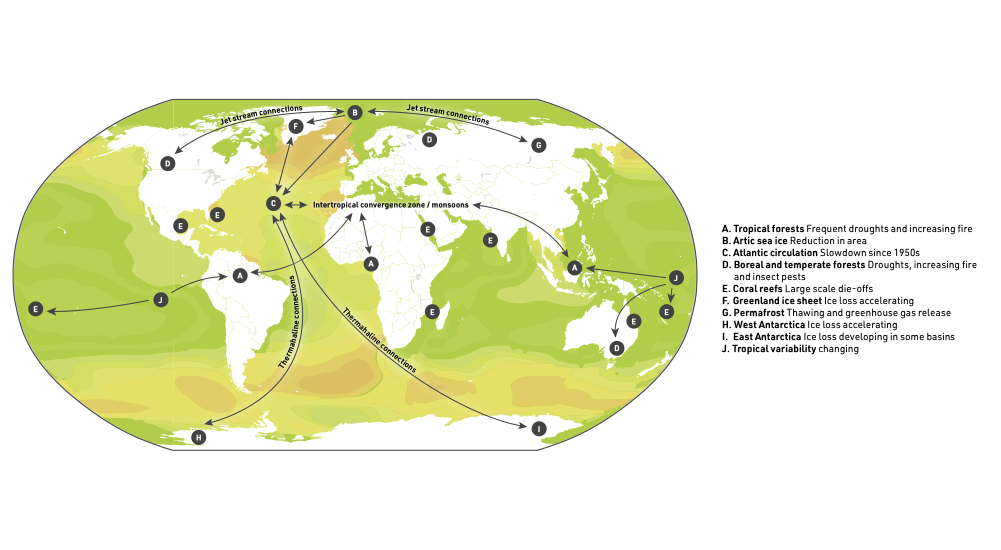
Figure 1. Map, adapted from Lenton et al. 2019 of tipping elements in the climate system. The background colours show ocean surface pH changes since the 1700s with greens indicating reductions in pH of around 0.06 and browns around 0.09 (see endnote)
Most discussion of tipping points focusses on the large-scale climate because these tipping points are globally connected with our climate system. Changes in ice sheets, for example, have global implications for sea level, and via changes in both ocean currents and the atmospheric circulation. The tipping points shown in Figure 1 cannot therefore be thought as “isolated” risks – all of them would have very significant global scale consequences. It is also very unlikely that we know of all of the important tipping points in the Earth system, particularly where these involve ecological tipping points in response to climate change.
What evidence do we have that tipping points exist?
There is no doubt that tipping points exist within the climate system – past climate records are full of examples of abrupt change and tipping points. Figure 2 below shows a few examples, including abrupt changes in ocean circulation, the abrupt onset of regional ocean deoxygenation, or fast and irreversible changes in vegetation when aridity passes a critical threshold. While past climate records provide evidence of the existence of some thresholds, they are not perfect analogues to predict the future. The most recent large-scale climate change on Earth – the transition out of the last glacial period around 20,000 years ago – was much colder than today, and therefore shows thresholds and tipping points characteristic of much colder climates than today. The last time the Earth was as warm as today, or even warmer, was hundreds of thousands to millions of years ago and the spatial and temporal resolution of paleoclimate proxies are too coarse for robust identification of tipping points that far back in Earth’s history.
Natural climate changes in Earth’s past were driven by the slow modulation in solar energy reaching the Earth associated with our orbit around the sun, or by natural changes in greenhouse gases in the atmosphere. These slow changes in climate forcings caused abrupt changes in some parts of the climate system through non-linear processes.
Today, human activities are causing concentrations of greenhouse gases in the atmosphere to rise significantly faster than the rate of any known natural change in Earth’s past.
Although human-caused climate change is very different to natural changes in the past – both in terms of the speed and magnitude of warming – we know that tipping points are a characteristic of how climate changes on Earth play out and so we shouldn’t ignore the very real risk posed by tipping points in the future.
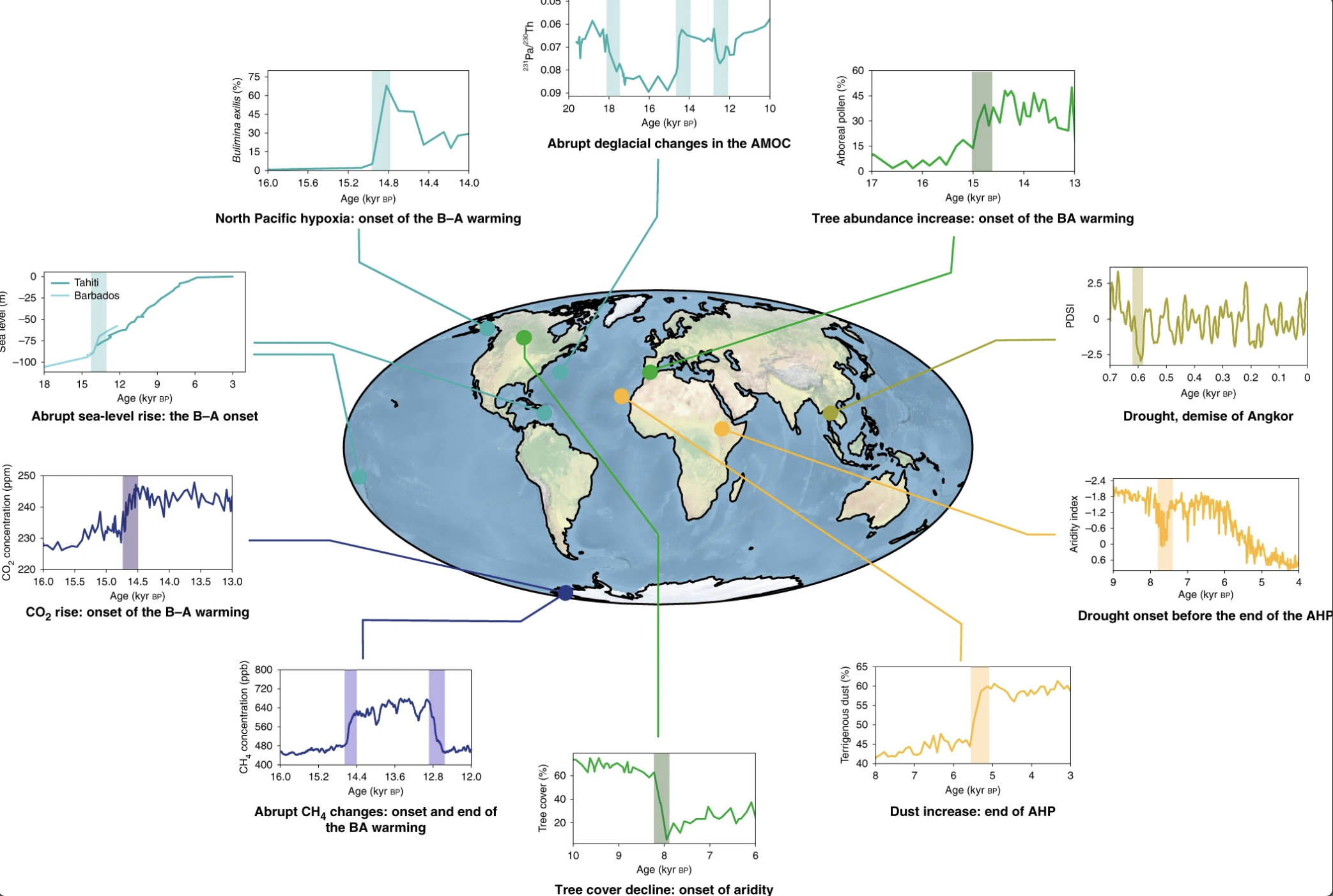
Climate change today and implications for tipping points
Since the beginning of the Industrial Revolution, the atmospheric CO2 concentration has risen from around 280 parts per million (ppm) to the current level of 417 ppm. Human activities have already caused Earth’s surface to warm by ~1.1°C relative to preindustrial times, leading to observable and worsening impacts through sea level rise, heatwaves, extreme rainfall, drought, fire and so on.
Climate warming does not progress in a smooth or linear manner, year-on-year. Rather warming occurs rapidly separated by periods of slow increases in a stair-case like way. One might think of these as periods of relative stability where negative feedbacks dominate to hold the system in quasi-equilibrium under increasing stress, until a threshold is reached and positive feedbacks dominate until a new relatively stable quasi-equilibrium is reached. Our problem, as humans, is that our economic system is optimised to the current climate; ports are built for current sea level, shipping is optimised to avoid cyclones, infrastructure is built with the current climate in mind and our agriculture is selected to provide food and fibre at a profit most years. Any change in climate modifies the optimisation point, changes the risk profile and requires investment merely to return to the previous optimal state.
Of the fifteen non-linear tipping points identified (Figure 1) around the world, all are at risk at being initiated by human-caused climate change over the coming decades to centuries. Today, the Greenland and Antarctic ice sheets are losing mass and significantly raising global sea levels. Recent observations of the strength of the ocean overturning circulation in the Atlantic seem to point to a weakening, that is outside of the range of normal variability over past millennia. Rapid sea ice loss in the Arctic is causing this region to warm at more than 3-times the global average, and permafrost thaw in the Arctic is adding to greenhouse gases in the atmosphere. Droughts and ecological shifts in the Amazon point to the ecosystem being more vulnerable than first thought. Increasing CO2 concentrations are leading to ocean acidification and deoxygenation. Heatwaves are threatening marine ecosystems, including widespread bleaching and mortality of coral reefs, and the combination of climate related pressures are potentially threatening ecosystems in many regions.
In each of these cases we do not know exactly how close we are to a tipping point, or even whether we have already passed it. We also do not always know if the changes are reversible, and if so, on what timescales. What is clear, however, is that the risk of passing multiple and possibly irreversible tipping points increases with each fraction of a degree of additional climate warming.
In the context of the future, there are tipping points that while not yet triggered may already be fully committed to. For example, the warming required for the West Antarctic Ice Sheet to permanently melt might have already been reached. This would ultimately lead to a 3 metre sea level rise that cannot be avoided even if global climate action keeps warming to below 2°C. There is evidence that the East Antarctic and Greenland ice sheets require sustained global warming of 3°C–5°C to be lost entirely1. Although irreversible on timescales of interest to humanity, the melting of these ice sheets will be much slower than the disintegration of the West Antarctic Ice Sheet.
Other tipping points may also be triggered between 1 – 2°C of global warming. Tipping points involving carbon cycle feedbacks (e.g. loss of the Amazon forest, loss of permafrost) can potentially add extra CO2 and methane (CH4) to the atmosphere, in addition to human emissions. This would reduce the available carbon budget for limiting warming in line with the 1.5°C – 2°C ambition of the Paris Agreement. However, for global warming up to 2°C above preindustrial levels, paleoclimate records indicate that the risk of amplification, such as runaway greenhouse gas feedbacks, appears to be small1,. It is very unlikely that tipping points in methane stored as gas clathrates in deeper terrestrial permafrost and subsea reservoirs will be triggered this century1. There is also potential for abrupt water cycle changes in the future1, but there is no overall consistency regarding the magnitude and timing of such changes. The Amazon is vulnerable to droughts, but also has some resilience to climate change in part due to its physical scale; deforestation likely reduces this resilience. The point at which the combination of climate change and deforestation forces the Amazon to cross a tipping point is not known with confidence1.
Some tipping points in the ocean are already evident. Collapse of coral reef systems associated with bleaching due to ocean warming appears to be inevitable, given these systems are already at very high risk and there is significant further warming still to be realized in the ocean. Complete collapse of overturning circulation in the Atlantic is not expected this century. Warming of 1.5°C, 2.0°C, or 3.0°C relative to preindustrial would probably see the thermohaline circulation continue to weaken for several decades by about 15%, 20% and 30% of its strength and then recover to pre-decline values over several centuries1. Additional marine ecosystem tipping points associated with ocean acidification and ocean deoxygenation are not well understood, although in many cases these stressors will be acting in conjunction with thermal stress.
The risk from tipping points can be viewed from two perspectives:
(a) the actual risk of a particular tipping point occurring this century may not be known with certainty. By analogy, we know for certain that a very major earthquake will hit the west coast of the US but we do not know if it will occur this year, this decade, this century or next century. It is the same with most tipping points. For example, we know that an equilibrium climate under current temperatures would have a sea level several meters higher than what we have today (likely 5–10m higher). We also know that an equilibrium climate under current CO2 concentrations would have a sea level 5–25m higher. So we know that this is where we are heading, we just don’t know how fast these systems will adjust and how “jumpy” they will be in making that adjustment. In other words, we know tipping points exist but we do not know exactly when they will be triggered.
(b) the actual risk of any tipping point occurring this century is non-zero. Most systems that undergo a tipping point are irreversible on millennial-scales – once triggered there is no going back. From this perspective humans need to avoid any tipping point from being triggered as an unambiguous priority, because the consequences would be overwhelming and potentially trigger cascading impacts that would represent existential risks to many people – and in some cases entire countries.
So far, the risk of tipping points has not been adequately incorporated into planning for climate change. The evidence for this is clear; if it was included in the Paris Agreement, and the more recent discussions in Glasgow, we would have agreed to much more aggressive action to avoid warming that increases the risk of tipping points being triggered. That is, the full implications of tipping points and the risks they pose are not accommodated in current thinking on reductions to emissions. The existence of tipping points is a key reason why nations need to cut emissions to limit warming to as close to a 1.5oC as possible.
How can we predict tipping points?
It may not be possible to precisely forecast tipping points, the level of CO2 that leads to tipping points, or how much warming can occur before a tipping point is triggered. However, in the same way as epidemiologists have warned for decades of an impending global pandemic without being able to accurately forecast the year, climate scientists can also make useful predictions concerning tipping points.
Anticipating abrupt changes in coupled climate, social, economic and ecological systems is crucial. To better predict such events, scientists can search for quantitative indicators that a system is nearing a tipping point. This approach closely investigates the behaviour of the system just before the change, “hindcasting” the change we know has happened. For example, increased autocorrelation in oxygen isotope records from Chinese caves is an indicator of shifts in east Asian monsoon activity. Similar early warning signals have been identified for the end of the African Humid Periods that led to a sudden aridification of the then “green” Sahara, or the onset of Dansgaard Oeschger events that saw rapid and significant temperature shifts in the Northern Hemisphere. It is possible that systematic analysis of networks of paleoclimate reconstructions could identify other characteristic early warning signs of tipping points. Since the rate of warming is now unprecedented, and the Earth is now anomalously warm, it is not possible to determine whether early warning signs from paleoclimate data are useful in terms of forecasting future events, but these past examples do inform our understanding of, and ability to model, tipping points.
Another approach is to use numerical representations of the whole three-dimensional complexity of the climate-carbon cycle system to “forecast” the risk of abrupt change. State-of-the-art climate models are based on the physics of the atmosphere and ocean, and include processes to project changes in temperature, precipitation, winds and ocean circulation. They are useful tools for the risk assessment of some of the known tipping points (such as ocean circulation changes, shifts in monsoons) even though they cannot replicate the full cascading effects of these tipping points. However, they lack some crucial components to be able to assess other tipping points (such as permafrost thaw, ice sheet melting, carbon cycle feedbacks). The recent development of Earth System Models is starting to fill this gap, by including ecosystems, carbon and nutrient cycles, and ice sheet processes into climate models. These Earth System Models are capable of simulating some abrupt changes, however, all climate models and Earth System Models still struggle to simulate the magnitude of some well-documented past abrupt events.
There are important limitations to overcome. Some are due to our limited understanding of critical processes, such as marine ecosystem dynamics and their impact on the marine carbon cycle. Others are due to computational power. For example, some tipping points are sensitive to regional-scale weather phenomena (e.g. Greenland melting has been accelerated by some extraordinary weather associated with changes in jet stream positioning in the last decade). We therefore need the weather-scale mechanisms and processes associated with tipping points to be integrated with our best high resolution climate models. This is beyond the research community’s current computational capability and points to the need for a reorganization of climate modelling science to inform policymakers on risk. A major step forward would see the integration of the mechanisms that are known to trigger tipping points into high resolution climate models, coupled with efforts to enhance the computational efficiency of these tools.
In summary, there are two connected strategies to better quantify the risk of tipping points:
(a) Paleoclimate data that enables processes, timing, and impacts of abrupt climate changes are valuable. They help establish some elements of risk, they provide process understanding, and they allow researchers to thoroughly assess the ability of climate models to incorporate known tipping points. Strategic collection of further data helps build our understanding of the earth system.
(b) Our climate and earth system models need to include the mechanisms that trigger and amplify abrupt change; the models can then be used to ask how much the risk of tipping points being triggered increases under different future greenhouse gas emission scenarios, and how much more probable it would be to trigger particular tipping points in the next few decades relative to late in the 21st century under a high emissions future.
Tipping points are, by definition, unlikely to be predictable in the sense of “it will happen in 2035” or any other specific year. Instead, we need to establish the evolving probability of a major event such as an ice sheet collapse, a coral reef system failure or a large-scale ecosystem die-back under increasing global warming. By establishing event probabilities, the magnitude of the threat can be established, and mitigation plans implemented to the extent possible. This can then be used, combined with risks of multiple tipping points, within economic analysis.
Ultimately, while tipping points are hard to predict, they are possible to identify and it is possible to indicate the conditions where they might be more likely to occur. Good long term data and improved modelling capabilities will tell us something about the scale of increasing risk over time, to plan for the major risks that would come with triggering tipping points. Ultimately though, risk planning should recognise the extreme risk that tipping points pose and guide decisions that limit the chance to initiating these abrupt and irreversible changes.
In summary, tipping points are not a theoretical possibility; they represent a real risk that is a serious threat to the habitability of our planet. The risks are not well understood, which warrants far more research. However, the single most urgent issue is to deeply cut greenhouse gas emissions so that we do not discover the consequences of triggering potentially catastrophic and irreversible outcomes.
References
View references in full in the PDF version of this briefing note available here for download.