We know land-sea breezes influence offshore precipitation throughout the tropics, but there is a dearth of direct observational data over oceans, so it is hard to understand how these breezes evolve throughout the day. CLEX researchers therefore combined data from four satellite scatterometer instruments to resolve land-sea breezes over the seas of the “Maritime Continent” (Northern Australia, Indonesia, Malaysia, and New Guinea.)
Satellite scatterometers are remarkable instruments which estimate surface winds over oceans by emitting radiation from space, then measuring the reflected radiation of centimetre scale ocean waves.
Land-sea breezes are typically thought of as coastal phenomena that arise from density differences between air over sea, and air over land, with the denser air slowly spreading out like a dollop of honey spreading out over a table. While this is true, our results show that these breezes also behave as waves, and can travel over 600 km away from coasts. These waves are examples of atmospheric gravity waves, which should not be confused with the gravitational waves detected in 2016 at the Laser Interferometer Gravitational-Wave Observatory (LIGO).
While gravitational waves are a consequence of modern physics (Einstein’s theory of general relativity) and involve ripples in the structure of spacetime itself, atmospheric gravity waves are a phenomena firmly rooted in classical physics, and are much more akin to the waves we see moving across the surface of the ocean. Ocean waves occur when one section of water is higher than another: we say that the higher section of water has negative buoyancy, because the water is much denser and heavier than the air horizontally adjacent to it. Gravity then attempts to “level out” this higher section of water, but this “levelling out” force – or restoring force – works too hard, pushing the raised section of water down but an adjacent section of water up. It’s this continual process that generates the ocean wave.
Atmospheric gravity waves have some similarities to ocean surface waves: in both cases the restoring force is gravity. However, with ocean surface waves there is a clear interface between the denser fluid (ocean water) and the lighter gas (air), and the wave motion is clearly visible at this interface. In the atmosphere things are more complicated as there is no such interface, and density, pressure and temperature all vary smoothly with height. This means that sometimes the atmosphere is unstable, and a section of air that is pushed upwards will continue to move upwards (this is how puffy cumulus clouds to form.) At other times the atmosphere is stable, and a section of air that is raised upwards will be forced down again by gravity, and wave motion can therefore be generated in a similar fashion to with ocean surface waves.
What is challenging to understand about atmospheric gravity waves, is that unlike ocean surface waves, they can also travel vertically. The GIF below provides an animation of Richard Rotunno’s 1983 solution to the set of equations describing an idealised two dimensional model of the tropical land-sea breeze. This solution was the first time atmospheric gravity wave behaviour of the land-sea breeze was exhibited in theory. The animation shows a horizontal cross-section of an idealised coastline, with the x-axis giving the distance from the coastline and the y-axis giving the height above the surface. Positive values of the x-axis can be thought of as land, and negative values as sea (imagine you’re at the beach watching the ocean, standing where the water is just reaching your feet, then you turn 90 degrees to your right.) One full cycle of the animation represents one day, and the arrows and shading give the direction and speed of the winds respectively.
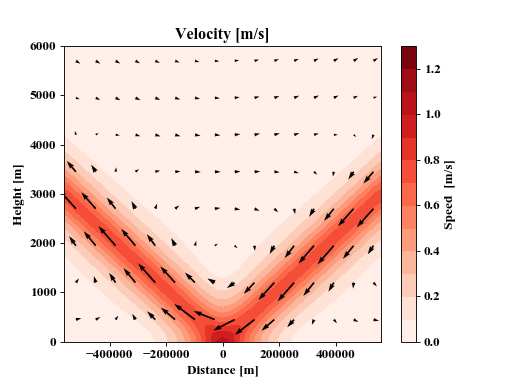
Our study used satellite scatterometry to measure the horizontal component of the winds over the sea surface (analogous to x<0, y=0 in the above animation). Although it was not the first study to do so – see in particular the pioneering work of Sarah Gille – by developing new ways of combining data from multiple scatterometer instruments it provided additional insight into the gravity wave structure of the land-sea breeze throughout the Maritime Continent, and how these waves relate to daily patterns of rainfall. Improving our understanding of these processes it crucial to our ability to improve how weather and climate models simulate precipitation in the tropics.
Planned future work will assess the vertical structure of these gravity waves using a series of weather balloon observations carried out during the upcoming “Year of the Maritime Continent” field campaign.
- Paper: Short, E., Vincent, C. L., Lane, T. P. Diurnal Cycle of Surface Winds in the Maritime Continent observed through Satellite Scatterometry. Monthly Weather Review. https://doi.org/10.1175/MWR-D-18-0433.1