- Detection determines whether a change in the climate is outside the historically expected range.
- Attribution investigates the causes of detected changes in the climate.
- We can use detection and attribution methods to understand our future risks.
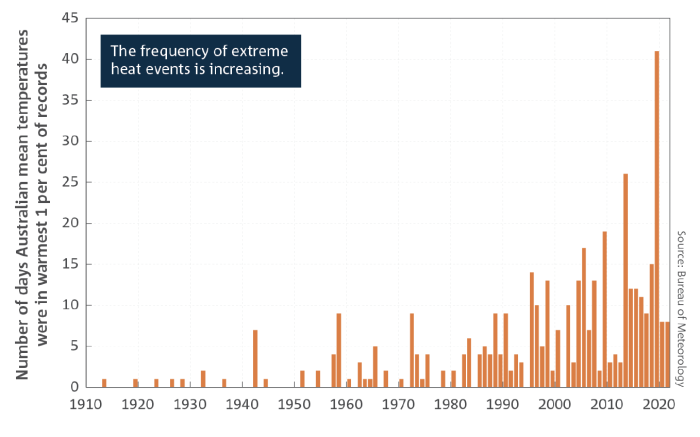
Detection and attribution science can be used to establish how anthropogenic climate change is impacting the climate. It is the process in which we identify or ‘detect’ any changes in weather and climate, and assign or ‘attribute’ the changes to various causes. Understanding the causes of climate extremes, and how they are impacted by climate change is key to assessing future climate risks. Detection and attribution research has the potential to inform a wide range of areas including climate change adaptation and mitigation policy, and disaster management and response.
What is detection?
Detection is the process to determine whether a change in the climate is outside the historically expected range.
It is normal for the climate to vary from year to year due to natural variability. However, detection involves identifying a trend that is outside what we expect due to natural variations.
For example, the number of days reaching extreme temperatures has been increasing in Australia since 1950 (Figure 1). For 2019, there were 33 days with temperatures over 39°C, exceeding the total number of days over 39°C which occurred across the whole 59 year period from 1960–2018. This is a detected increase which has been unprecedented in the historical period.
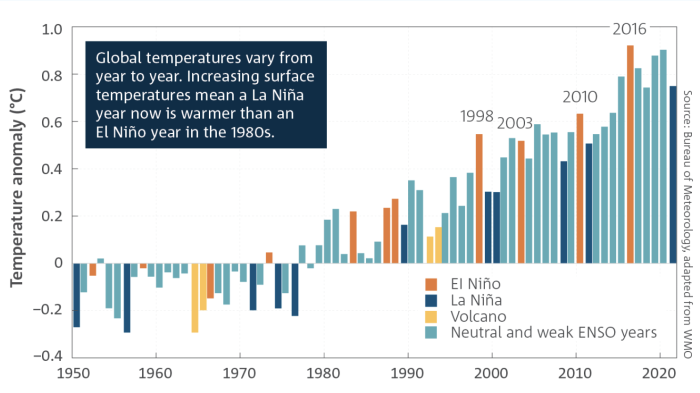
Natural variability
There are many different processes that interact to influence the climate we experience. Natural variability refers to changes in the climate that occur due to processes other than human influence. These include processes such as large-scale climate modes like the El Niño-Southern Oscillation (ENSO), volcanic eruptions which can have a cooling effect on the climate, or small changes in the energy received by the Earth’s surface from the sun.
Natural variability can lead to large year to year differences in our climate. For example, global temperatures are often higher during El Niño events when heat is released from the ocean to the atmosphere.
La Niña years are typically cooler. This cycle means that the increase in global temperatures rise more like a staircase rather than a straight line.
Figure 2 demonstrates how year to year differences in temperature are caused by natural variability, but human induced climate change causes the overall long term upward trend.
Natural variability’s influence on the climate is important when looking at shorter time scales such as 1 year to 20 years. On longer timescales, the influence of natural variability is minimal compared to human impacts.
Looking across the 1950 – 2022 period in Figure 2, the warming trend caused by human impacts becomes evident.
What is attribution?
Attribution is the process where the causes of detected changes in the climate are determined.
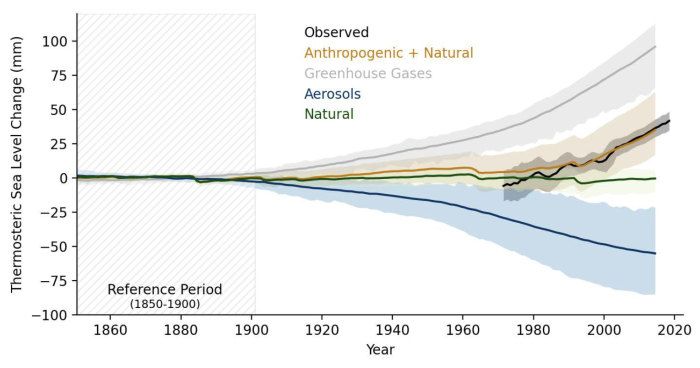
The causes are known as climate forcings and may be natural (e.g. changes in the solar cycle, volcanic eruptions, La Niña) or human (e.g. emissions of greenhouse gases, deforestation, aerosols).
Two key types of attribution include:
- attribution of climate variables
- attribution of climate events.
Here we focus on the attribution of climate variables and the process involved in the attribution of a variable.
Attribution of climate variables involves determining the cause of a detected change in a climate variable, such as temperature, precipitation, ocean heat content or sea-level, over a time period. This is often from a pre-industrial period (defined as 1850-1900 in Figure 3) to the present day.
Attribution of climate variables allows us to determine what causes an observed trend in a variable. For example, it is very likely that human influence caused an increase in the global mean sea level (with contributions from sources including melting glaciers, ice sheets and warming oceans) by 0.20m between 1901 and 2018.
The history of detection and attribution
Detection and attribution science began with the theory that the composition of the atmosphere can impact the climate. This idea goes back more than a century.
Before formal methods of detection and attribution existed, scientists were aware that the climate system was sensitive to variations in the amount of energy received from the sun and how that was distributed across the land, ocean and atmosphere and back out into space.
In 1856, experiments by the pioneering American scientist, Eunice Newton Foote found that CO2 absorbs heat. She concluded that an atmosphere with higher concentrations of CO2 would cause Earth’s temperature to increase. This is known as the greenhouse effect, where greenhouse gases warm the climate.
Formal methods of detection and attribution, known as ‘fingerprinting’ were first established in the 1990s. Fingerprinting was used to identify the causes of the observed warming trend in global temperatures and informed the Intergovernmental Panel on Climate Change’s (IPCC) Second Assessment Report in 1995.
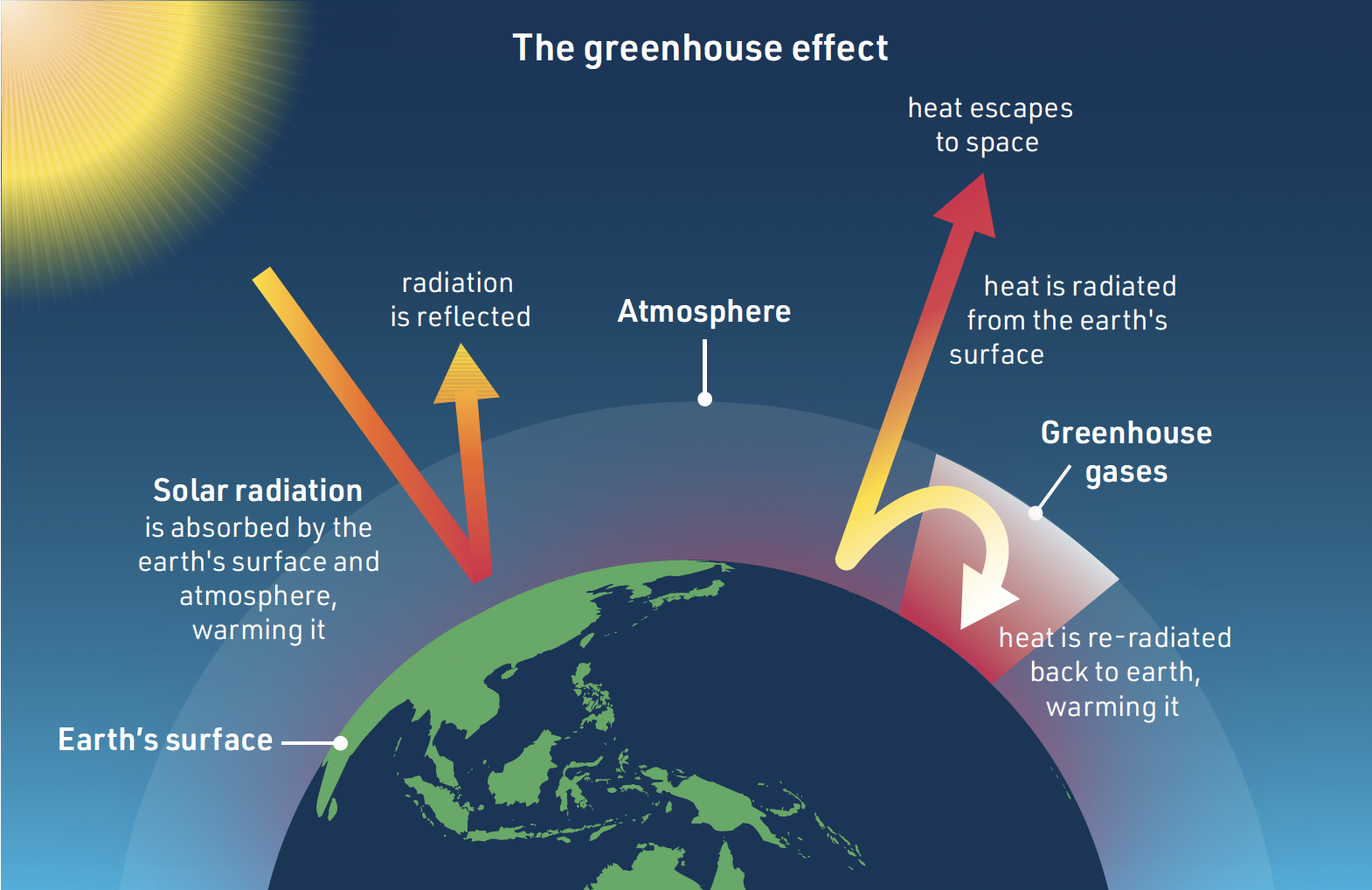
Figure 4: The greenhouse effect is the warming of the earth’s surface caused by greenhouse gases in the atmosphere, which interrupt the radiation of heat away from earth and back out to space. When radiation leaving earth hits greenhouse gases in the atmosphere, the gases cause it to re-radiate in all directions, with some of it heading back to earth causing warming. Source: ARC Centre of Excellence for Climate Extremes.
The report concluded that “the balance of evidence suggests a discernible human influence on global climate”. The report noted that to achieve greater confidence, more evidence was required. Across each subsequent IPCC report (2001, 2005, 2009, 2013), confidence in the impact of anthropogenic greenhouse gases on climate change has increased, with improved models, better observational and paleoclimate datasets and new techniques in detection and attribution. By the Sixth Assessment Report in 2021, the IPCC declared:
“it is unequivocal that human influence has warmed the atmosphere, ocean and land.”
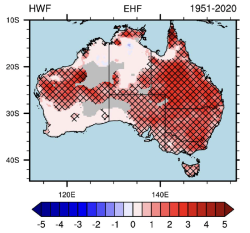
The science of detection and attribution has progressed to look at changes in variables other than temperature, including rainfall, snow and ice cover, storms, cyclones and wildfires. Detection and attribution now looks at regional and local scales, as well as individual extreme events. These methods continue to advance and the ARC Centre of Excellence for Climate Extremes is actively contributing to the evolution of detection and attribution science.
How is detection and attribution possible?
Usually, three elements are required for a robust detection and attribution study:
- High quality observational data over adequate spatial and time scales (needed for detection).
- An understanding of the physical processes behind the climate variable of interest (needed for attribution).
- The ability to model the climate variable, underpinning processes, and the observed changes (needed for attribution).
How do we detect a change in the climate?
High quality observational data over adequate spatial and time scales is essential for detecting whether a change in the climate has occurred. To detect a trend, scientists examine the observational record of the variable in question and assess whether significant changes have occurred. This involves comparing recent observations to an earlier time period, or calculating a trend over the whole time period.
Statistical analysis is used to determine whether there has been a statistically significant change in the variable over the time period in question i.e. a change that cannot be explained by chance alone. The choice of statistical tests is chosen based on the variable in question. Statistical analysis allows us to determine if:
- a statistically significant change (not explainable by chance alone) in a climate variable has occurred; or
- there is no significant change and so natural variations in the climate cannot be ruled out.
For example, recent research by the Centre detected that heatwave trends have been increasing across most of Australia since 1951 (Figure 5). This study used high quality observational data over Australia and analysed temperature over the 1951-2020 time period to understand how heatwaves have changed. The study detected statistically significant trends across much of Australia, concluding that heatwaves are intensifying, becoming hotter, longer and more frequent. Figure 5 shows areas where the number of heatwave days has been increasing in red, with the black hatching indicating statistically significant trends (i.e. unlikely to be due to chance).
How do we attribute a change in the climate?
We use climate models to understand more about what causes changes in our climate. Climate models allow us to run experiments where we can study the climate with or without potential causal factors present and see which factors reproduce our observations.
The first step is to evaluate:
Do we have a good understanding of the physical processes that impact the climate variable in question?
If climate models do not simulate processes realistically, they cannot provide reliable information on the processes driving the variable.
Can climate models simulate this process accurately?
If the model simulates the process of interest well, we can proceed to analyse further simulations. This generally involves running two types of model experiments over the recent historical period (say the last 100-200 years):
- one which includes natural variability and human impacts, accounting for greenhouse gases, aerosols and land use changes (the ‘world that was’) and
- one which includes only natural variability which demonstrates the climate we would expect to occur without human impacts (the ‘world that might have been’).
Comparing the two experiments allows researchers to see how human activities have impacted the climate system. Researchers repeat the same experiment multiple times to account for the variability within the models themselves. The results are tested for statistical significance which allows us to formally attribute the observed changes to human activities.
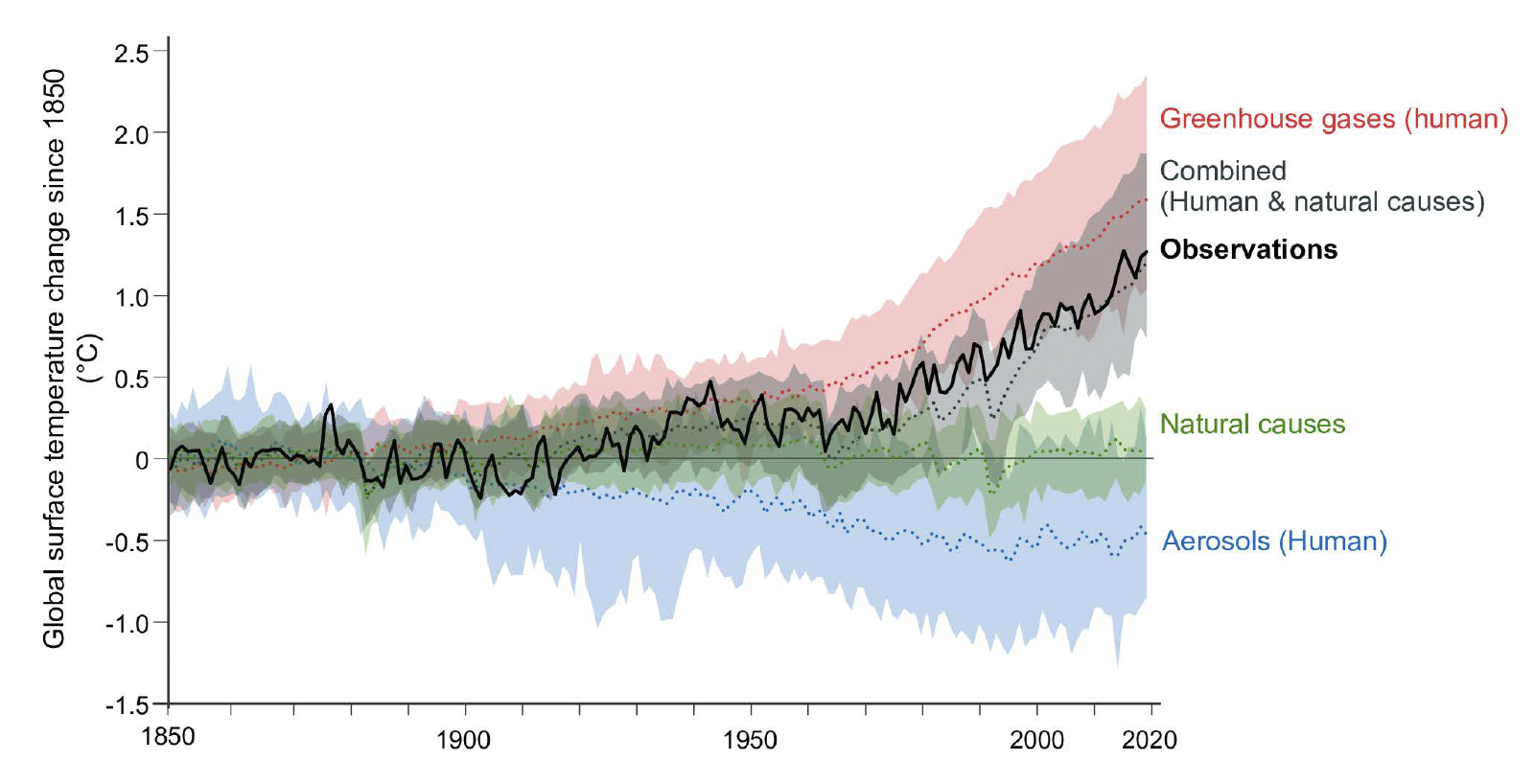
For example, Figure 6 shows the global surface temperature change since 1850 showing observations and model simulations:
- The grey band shows the model simulations of the response in global surface temperature to human and natural forcings.
- The red band shows the model simulations of the response in global surface temperature to greenhouse gases only.
- The blue band shows the model simulations of the response in global surface temperature to aerosols and other human drivers (except greenhouse gases).
- The green band shows the model simulations of the response in global surface temperature to natural forcings only.
- The solid black line shows the observations and the other coloured lines show the multi-model mean.
Fingerprinting, as described earlier, is used to quantify the influence of each human activity or ‘climate forcing’. We create experiments where we isolate individual factors (e.g. greenhouse gases, aerosols or land use change) to see how the climate responds. Each different human activity causes a distinct global warming pattern, or fingerprint. We can compare the fingerprint created by each human activity with observations. This will determine whether the fingerprint from that particular forcing is noticeable in the observations. This allows us to measure the impact of each forcing on the observed variable of interest.
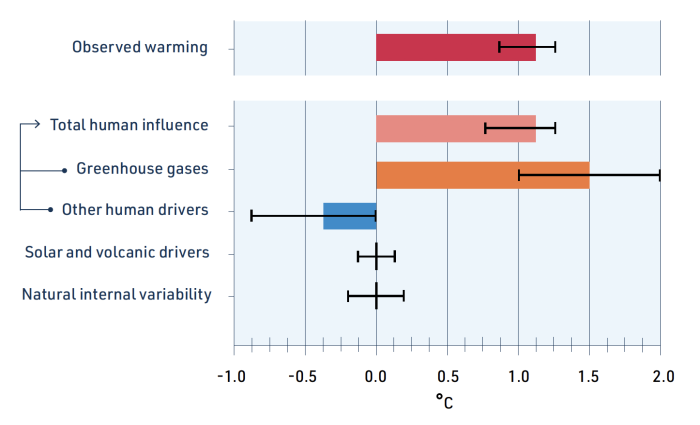
Figure 7 shows the contributions of different climate forcings to the observed global temperature increase since the pre-industrial period using fingerprinting methods.
Greenhouse gases have contributed to 1.5°C of warming while other human drivers (e.g. aerosols and land-use change) have caused 0.4°C of cooling.
This has resulted in the net warming of 1.1°C. Solar and volcanic drivers, as well as the natural internal variability of the climate system have not impacted the change in global temperature over this long time period.
It is easier to attribute temperature than other variables.
In general, we are more confident in attributing temperature to climate change than other variables. This is because we have a long record of high quality temperature observations covering most of the globe, heat extremes are well simulated by climate models and the physical processes around temperature are well understood.
In contrast, other extremes such as heavy rainfall are much harder to attribute as they:
- often occur on more local scales,
- are more intermittent,
- are more variable in time,
- are less well observed and
- are less well simulated by models.
Some phenomena, such as global trends in droughts or floods are the result of multiple processes occurring over varying periods of time. A drought can be influenced by many factors such as rainfall, evaporation, or changes in vegetation over timescales that might range from weeks to years. Trends in multifaceted phenomena can be hard to attribute to specific anthropogenic forcings and makes detection and attribution much more challenging.
How does detection and attribution relate to risk?
We can use detection and attribution methods to inform policymakers on future risks to humans and ecosystems under different scenarios of future climate change. Through detection and attribution research, it is unequivocal that human influence has warmed the atmosphere, ocean and land. Continued greenhouse gas emissions will cause further warming of the climate. We also know that the cooling effect caused by aerosols will decline over the coming decades, further adding to the warming. Other changes such as ocean warming, sea level rise, changes in rainfall extremes, drought and increased adverse fire weather have been detected. Understanding these trends provides information to anticipate future risks from climate change and plan adaptation.
As detection and attribution methods develop, our capacity to establish causal links to observations are improving. The field of extreme event attribution is evolving, allowing researchers to determine whether specific extreme events have or will become ‘more likely’, ‘more frequent’ and/or ‘more severe’ due to human caused climate change.
Various extreme event attribution studies are now assessing how climate change has influenced the impacts of a specific extreme event, such as health impacts or financial damages.
This extension of extreme event attribution is still in its infancy, with the potential to inform loss and damages in debate amongst the science community. However, further developments in this new field have great potential in aiding discussions on the risks or negative consequences of climate change.
Understanding the role of climate change behind costly or deadly impacts allows for mitigation and response systems such as building codes or public health resources to be sufficiently bolstered, particularly as the risk of impacts increases.
What is the ARC Centre of Excellence for Climate Extremes doing to advance attribution science?
The ARC Centre of Excellence for Climate Extremes undertakes fundamental research that improves our scientific understanding of physical processes and the capability of climate models to simulate the processes. The Centre is also developing methods such as machine learning to produce faster, cost effective results to expand the information from model experiments.
Recent research at the ARC Centre of Excellence for Climate Extremes has shown:
- The overall intensity of the 2017/18 Tasman Sea marine heatwave was virtually impossible without anthropogenic forcing.
- Global average marine heatwave frequency and duration has increased by 34% and 17% respectively, resulting in a 54% increase in annual marine heatwave days. Importantly, these trends can largely be explained by increases in mean ocean temperatures, suggesting that we can expect further increases in marine heatwave days under continued global warming.
- Rapid rain bursts have intensified over the past two decades by around 40% in Sydney. This is an unexpected rate of change beyond anything that has been seen before and is the first time this phenomenon has been clearly documented anywhere in the world. This trend could not be attributed to natural variability, leaving climate change as a possibility, but further research is necessary to pinpoint the exact cause of this trend.
- More than 50% of the land surface has experienced robust changes in these hydrological cycle components since 1980. Of particular concern is increasing water-resource stresses in key breadbasket regions, including in Australia, and in some densely populated areas. Using observations, data assimilation approaches and machine learning, the results support the general conclusion that over land “wet gets wetter but dry does not get drier”.
- Evapotranspiration (the water evaporating from the soil, land surface and through plant leaves) shows an increasing trend since 1980 over most of the earth’s surface.
- Currently the Centre is investigating the causes of the 2022 East Australian extreme rainfall events using observational analysis and high resolution climate models.
- The Centre is developing hypothetical experiments where we remove the influence of large-scale drivers like ENSO to understand teleconnections to Australian rainfall.
Briefing note created by Professor Julie Arblaster, Professor Lisa Alexander and Associate Professor Sarah Perkins-Kirkpatrick.
Reference list and author bios available in the PDF version below.