- Ocean biogeochemistry refers to the interactions between the ocean’s biological, geological and chemical processes.
- Our research advances the understanding of biogeochemistry in areas including ocean productivity, carbon cycling and eddies as well as high resolution ocean modelling.
- Our research improves projections of ocean states to understand the impacts of climate change on fisheries and marine life and to calculate current and future carbon budgets.
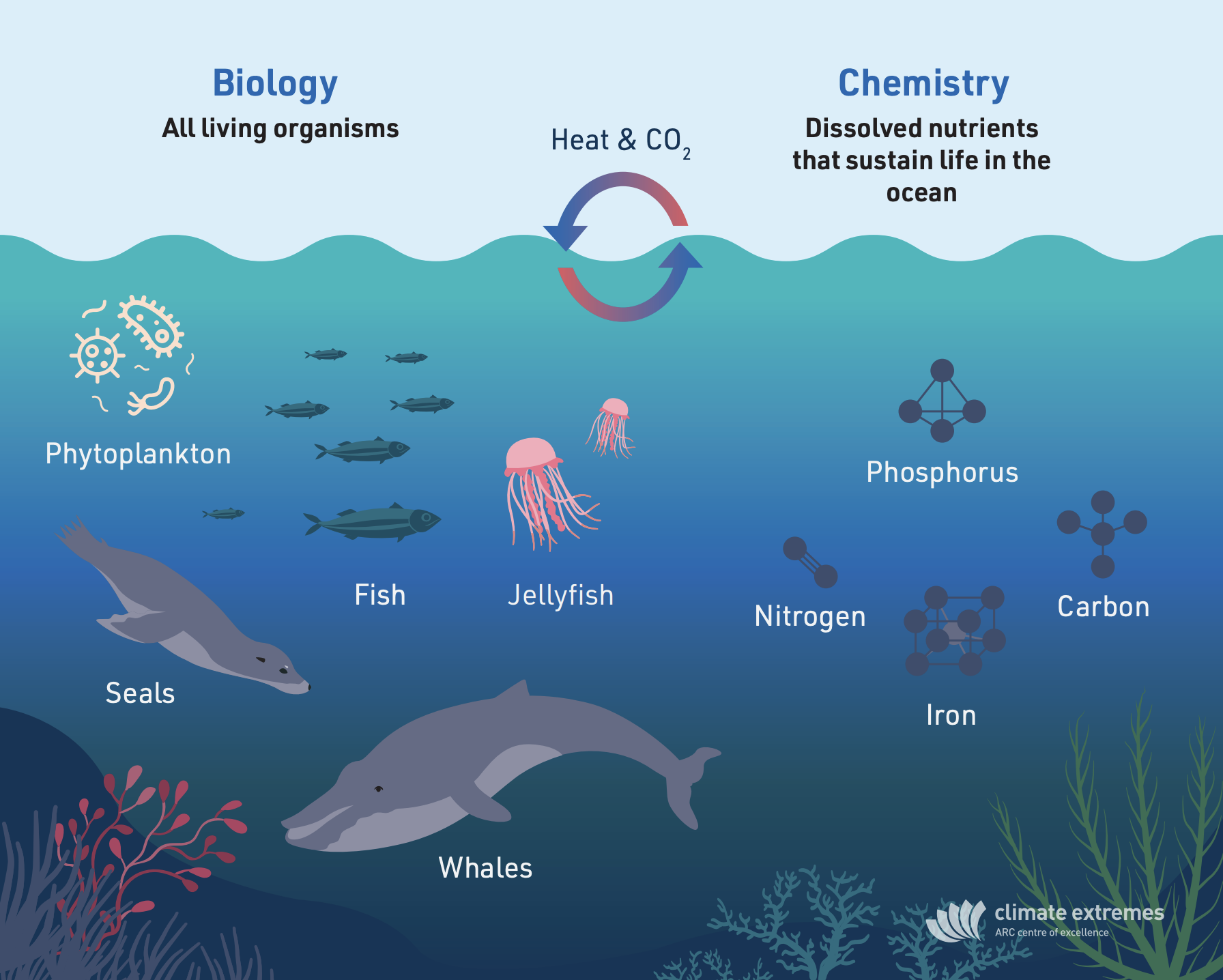
Ocean biogeochemistry refers to the interactions between the oceans’ biological, geological and chemical processes (Figure 1).
Ocean biology includes all living organisms from phytoplankton at the base of the food web, to fish, to large marine mammals such as whales.
Ocean chemistry refers to dissolved nutrients such as nitrogen, phosphorus, carbon and iron which sustain life in the ocean.
The oceans’ biology and chemistry influence the climate because ocean, atmospheric and land processes are all interconnected. An example of this is the transport of carbon from the atmosphere to the deep ocean through ocean surface productivity, subsurface chemical transformations and ocean circulation. This process is called the oceans’ ‘biological pump’ and affects the marine ecosystem which alters the chemistry and temperature of the atmosphere thereby impacting the broader climate.
How does ocean biogeochemistry impact climate change?
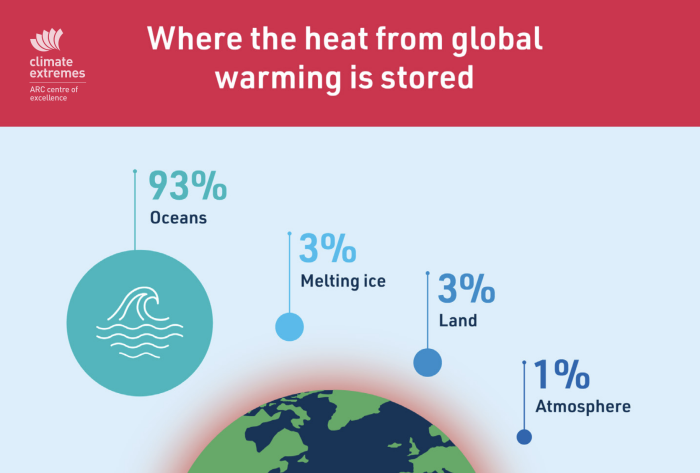
The ocean buffers the atmosphere to the impacts of climate change through the uptake of heat and carbon dioxide from anthropogenic emissions.
The oceans have absorbed more than 93% of the heat and approximately 26% of the carbon dioxide (CO2) from anthropogenic emissions.
This has helped limit warming of the atmosphere, but it has altered the chemistry of the ocean causing negative impacts on marine life and associated ecosystem services.
The important role of ocean biogeochemistry in regulating the broader climate can be seen from historical observations. Ice core records confirm that atmospheric CO2 was lower during the ice ages (glacial periods). Up to half of the change in atmospheric CO2 between past glacial and interglacial (warm) periods was due to changes in the strength of the oceans’ biological pump. That is, a more productive ocean and a stronger biological pump drove half of the CO2 difference between the last ice age and the pre-industrial period (~1800). The other half was likely due to changes in ocean circulation.
The oceans’ uptake of CO2 has implications for achieving net zero emissions. There is a limit to the amount of CO2 that the oceans can absorb, and by some estimates the ocean carbon sink will have reached its full capacity before 2100. This means the remaining anthropogenic emissions will have to be stored in the land, soil or atmosphere carbon sinks.
Improving our understanding of marine biogeochemical processes assists in quantifying ocean carbon storage.
Why is studying ocean biogeochemistry important?
Biogeochemistry controls marine and terrestrial ecosystems and impacts the climate we experience. The oceans are vast, over 3000 metres deep on average, and home to complex ecosystems. Large areas of the ocean are yet to be explored and there are still significant gaps in our understanding, including how ocean ecosystems interact with ocean biogeochemistry. The Intergovernmental Panel on Climate Change (IPCC) identified knowledge gaps in ocean biogeochemistry, including uncertainties in:
- patterns of primary productivity,
- oxygen content,
- acidification and
- the impacts of changing ocean circulation and mixing.
It is expected that the ocean will undergo significant changes this century, and these changes vary depending on the rate of greenhouse gas emissions in the future. The incomplete understanding of biogeochemical processes limits our ability to understand how they may change over time.
Two key areas of ocean biogeochemistry studied at the ARC Centre of Excellence for Climate Extremes include ocean primary productivity and the influence of eddies on biogeochemistry. The Centre is building an understanding of these processes to improve ocean modelling. This work helps to produce realistic projections of environmental change under future climate scenarios which inform risk assessments and adaptation strategies.
Understanding the physics and chemistry of primary productivity
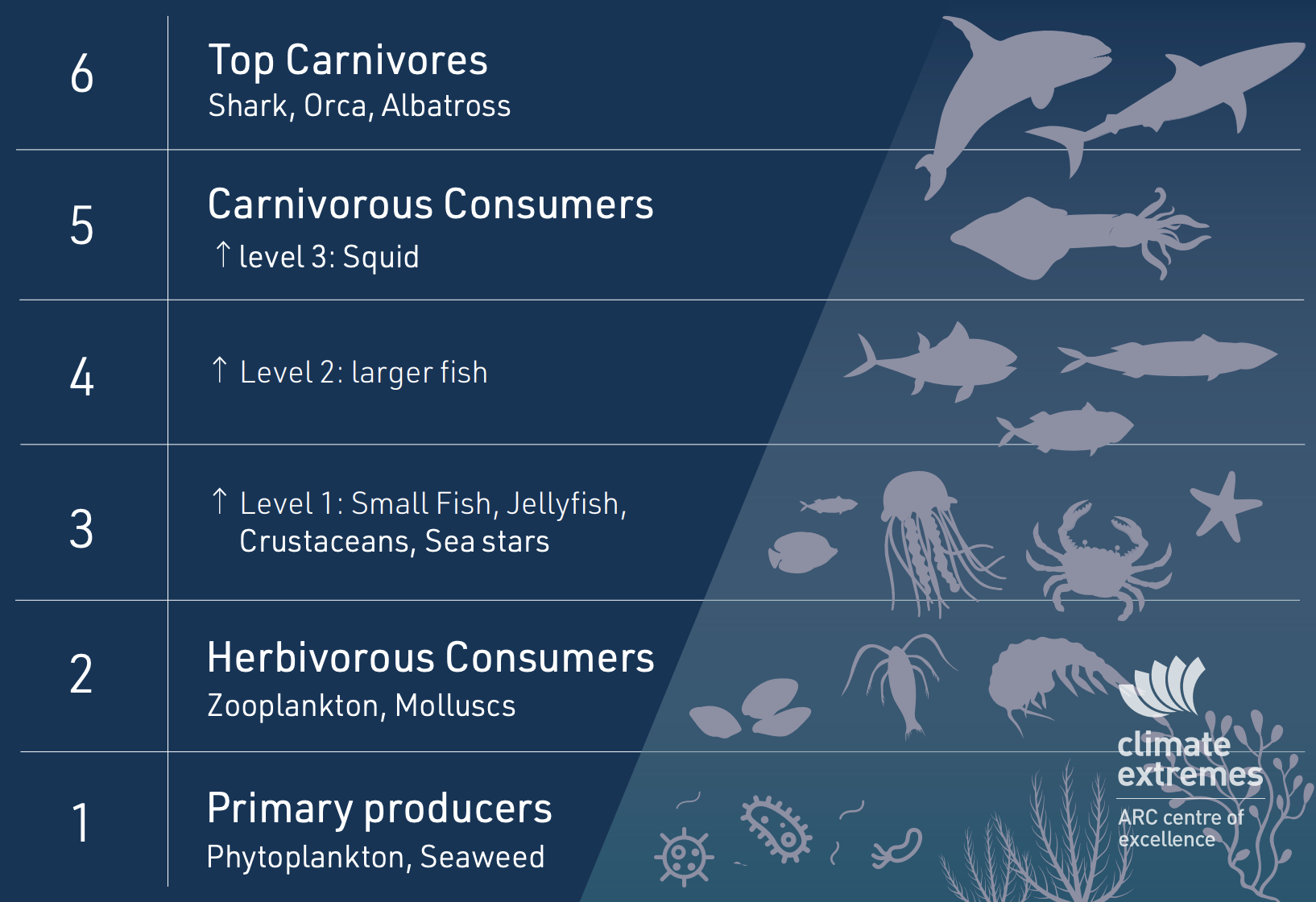
Primary productivity refers to the use of carbon and other nutrients by primary producers, such as phytoplankton, to grow and reproduce. Primary producers form the base of the marine food chain (Figure 2). Primary productivity facilitates the uptake of carbon by the oceans’ biological pump (Figure 3).
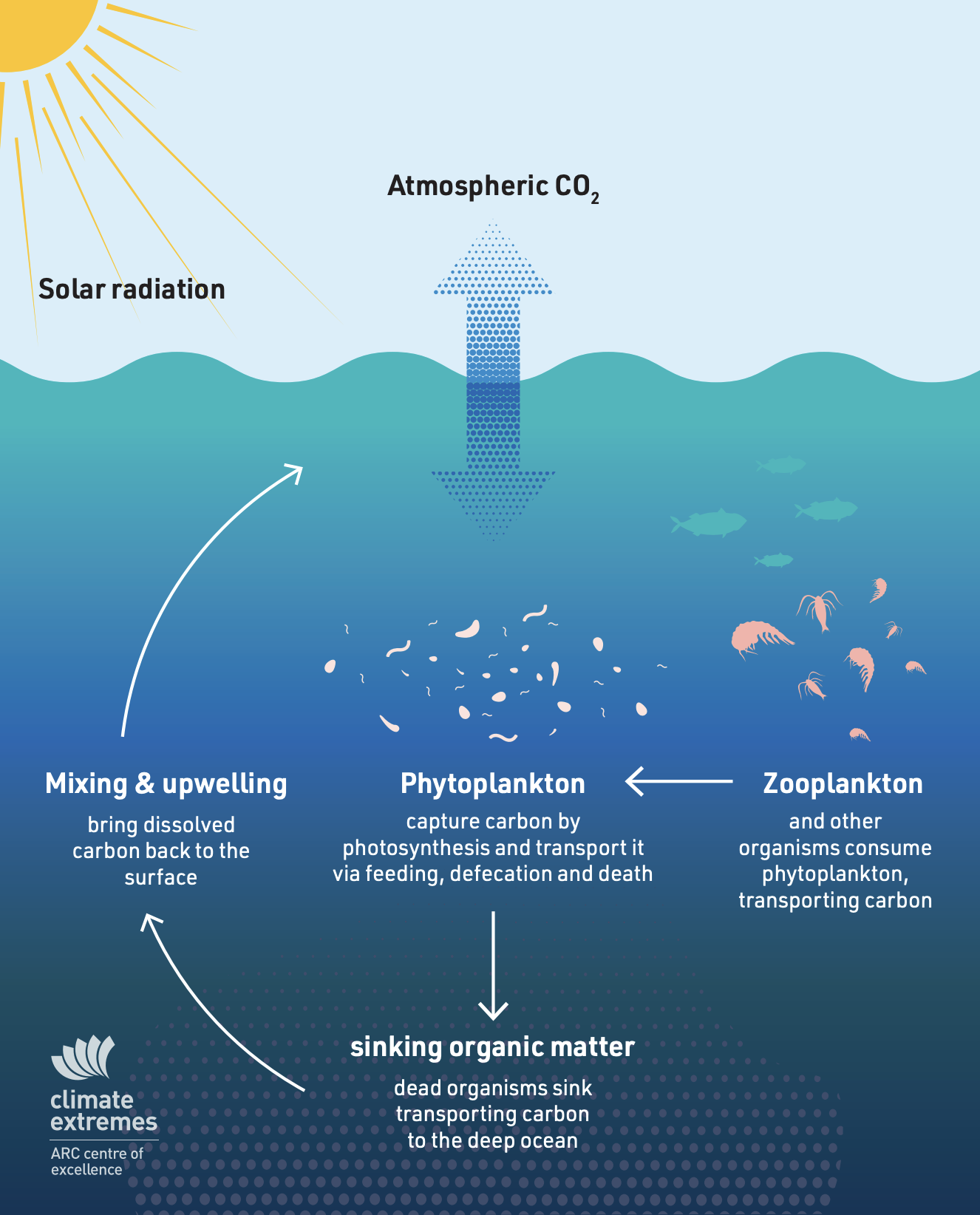
This process plays an important role in the exchange of CO2 in and out of the ocean shown in Figure 4. Regions where CO2 is being taken up by the ocean are places where ocean productivity is high or there is sinking of cold, dense water (blue and purple in Figure 4). Areas that are releasing CO2 to the atmosphere are locations where ocean productivity is low due to lack of nutrients, primarily dissolved iron (red and yellow in Figure 4).
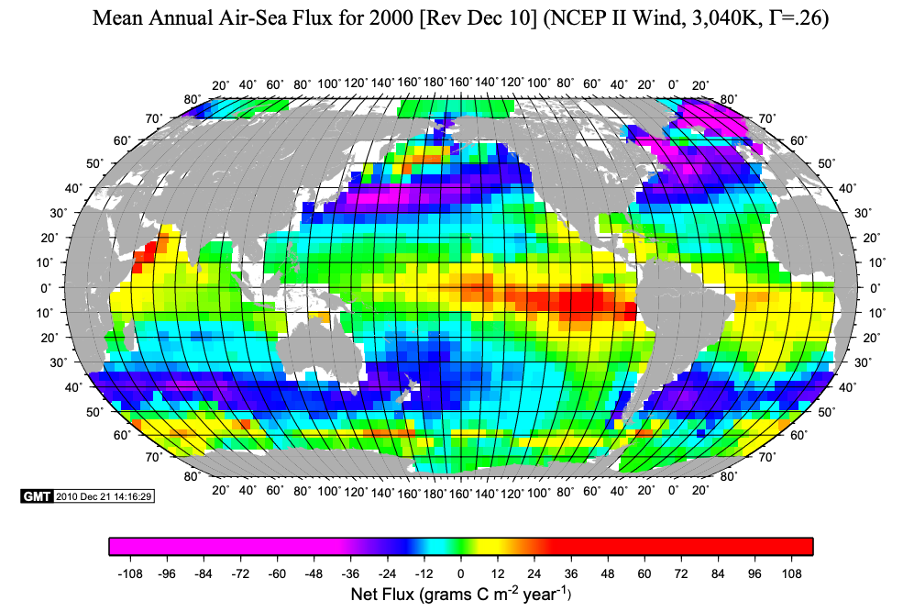
Figure 4: Annual average exchange of CO2 between the ocean and atmosphere. Blue/ purple shows ocean take up of atmospheric CO2, red/yellow shows ocean release of CO2 to the atmosphere. Source – Columbia Climate School.
The IPCC found there will be a significant decrease in net primary productivity under a high future emissions scenario. This would weaken the oceans’ biological carbon pump with implications for the entire marine ecosystem, including less productive fisheries.
Representing patterns of primary productivity is a current challenge for climate models, due to incomplete understanding of the drivers and historical trends of productivity. Research at the Centre is improving our understanding of the physics and chemistry of primary productivity and how this is likely to change in the future.
Recent work has used ship-board measurements to understand how light, nutrients and ocean-ice dynamics regulate productivity in Southern Ocean environments that are particularly susceptible to climate change.
The Centre has also investigated the links between Australian bushfires and ocean productivity, documenting the effects of aerosol deposition in the ocean.
On smaller spatial scales, our work has shown for the first time the productivity of ocean eddies under the water surface.
This was done using robotic Argo floats which can examine sub surface features. All of these studies, and other work by the Centre, help us to refine our estimates of the flux of CO2 into the ocean in Figure 4, and how it may change in the future.
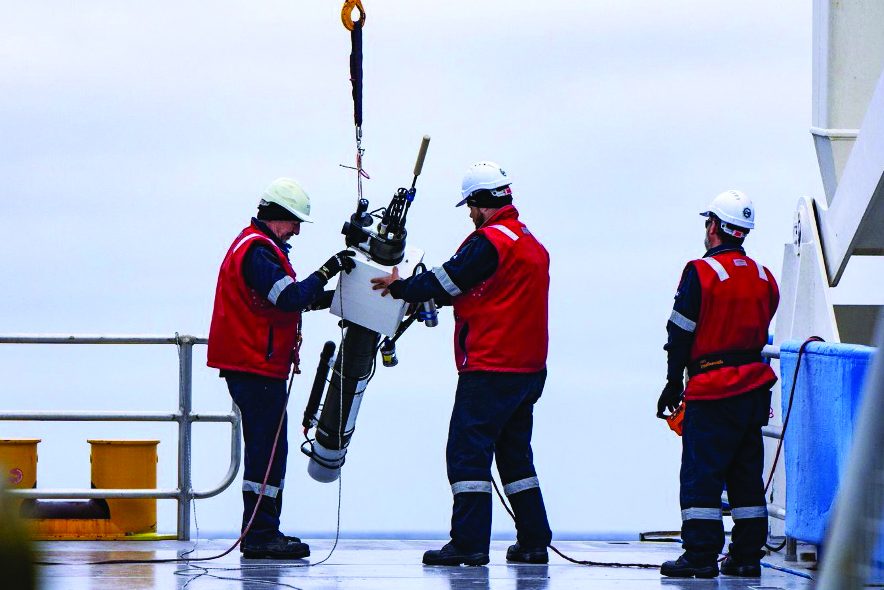
Argo float technology
The ARC Centre of Excellence for Climate Extremes uses data from Argo floats to study ocean biogeochemistry (BGC). BGC-Argo floats are robotic devices, released into the ocean to measure physical and biogeochemical properties. These include temperature, salinity, dissolved oxygen, light, chlorophyll, phytoplankton particles, dissolved nutrients (nitrate) and acidity (pH). The floats can adjust their buoyancy to move up and down through the upper 2,000 m of the ocean every 10 days. These floats are revolutionising the access to biogeochemical data, allowing scientists to monitor ecosystem health and important processes such as ocean warming, carbon uptake, acidification, deoxygenation and impacts on phytoplankton. Scientists can use the BGC-Argo data to validate satellite observations. The BGC-Argo community aspires to have a global fleet of 1,000 floats and the Centre is an active contributor to the new knowledge emerging from the global BGC-Argo array.
What impact do ocean eddies have on productivity?
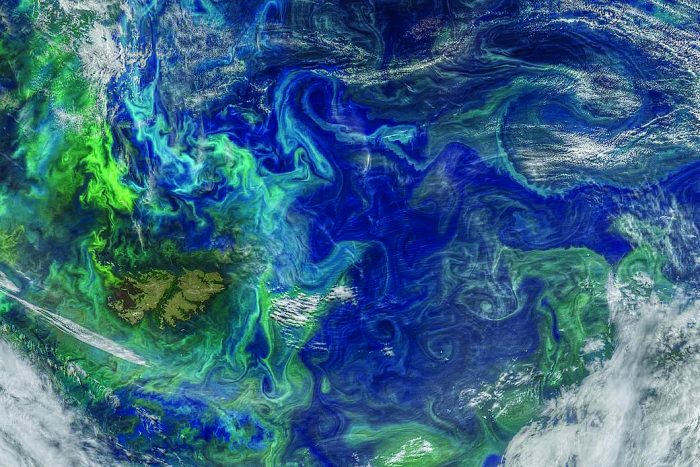
Ocean eddies are spinning parcels of water about 100 to 200 km across and at least 1,000m deep (Figure 5). Eddies have an important impact on biogeochemistry, influencing productivity and CO2 uptake by transporting heat, salt and nutrients in the ocean. At any given time, there are around 1,300 eddies in the Southern Ocean. Eddies are difficult to sample by traditional methods such as ships, as they are relatively short-lived and constantly moving. As a result, they are currently not well understood or accurately represented in climate models.
The Centre is using observational data from satellites, historical ship data and the growing BGC-Argo array, as well as high resolution models to improve our understanding of eddies. Recent work has found that the number of eddies and the energy they contain has increased in the Southern Ocean over recent decades.
This may increase the north-south transport of heat, salt and nutrients, or lead to more abundant hot-spots of ocean productivity, but we don’t know this until we are able to accurately represent these processes in models. Our current work is pursuing two important knowledge gaps in this area:
- Using ship data, BGC-Argo and satellites to develop techniques that map the nutrient content and productivity of Southern Ocean eddies. This will show their contribution to ocean productivity and the uptake of atmospheric CO2.
- Improving the representation of eddies in high resolution ocean models to quantify their past and future contribution to Southern Ocean carbon cycling and climate.
Modelling the ocean at high resolution
The ARC Centre of Excellence for Climate Extremes is at the forefront of Australian and international ocean modelling and is part of an active community which is improving the physics and biogeochemistry of Australia’s community climate model, called Australian Community Climate Earth System Simulator – a National Research Infrastructure (ACCESS-NRI). The Centre is also a member of the Consortium for Ocean-Sea Ice Modelling in Australia (COSIMA), which integrates the capabilities of different groups within Australia’s ocean & sea ice modelling community.
These efforts are improving our capabilities to model the ocean at high resolution. This allows us to understand and predict the importance of relatively small ocean phenomena including eddies. A suite of ocean processes can be represented in climate models: from currents and mixing to nutrients and carbon chemistry to phytoplankton productivity and fish. Modelling provides insights into the system which cannot persistently be measured at the appropriate time and space scales and provides a guide towards improved observational systems. Models allow us to simulate ocean conditions in the past and future and where we do not have measurements as well as hypothetical ocean states.
Perhaps most importantly, models allow us to perform experiments to understand the consequences of our actions (or inaction) for future climate.
Briefing note created by Peter Strutton and Ramkrushnbhai Patel.
Reference list and author bios available in the PDF version below.