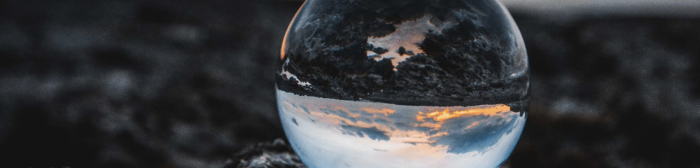
What is a climate model?
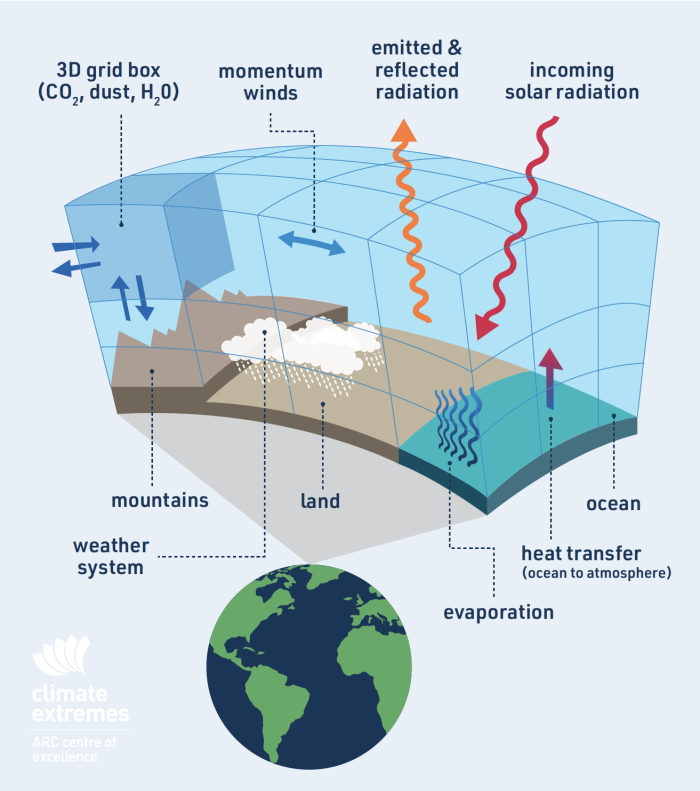
A climate model is a tool which directly applies the physical laws that govern our climate system to simulate the past, present, or future of our climate.
They differ from some types of modelling which use statistical extrapolations of present-day conditions. Physical laws are derived from fundamental physics, which govern the flow of air in the atmosphere, water in the ocean and heat and moisture in the land surface. These laws do not change, even as the climate changes. The laws can be expressed as mathematical equations which can be solved on a computer.
Solving these equations allows simulations of the past, present and future of our climate to be made, including simulations of temperature, rainfall, the atmosphere and ocean circulation, and soil moisture.
Climate processes and the physical laws which represent them are complex. Useful solutions can be found using techniques which divide the atmosphere and ocean into many layers in the vertical and split each layer into many grid elements (or grid squares) in the horizontal (Figure 1).
Imagine a 3-dimensional chess board where pieces, in this case parcels of air or ocean water, can move to any adjoining square. Within each three-dimensional square, computers can be used to estimate solutions to a large number of equations.
A finer grid makes for more precise solutions; however, it also makes for lots more grid boxes, which increases the computational cost taking more time to complete. Therefore, a trade-off between the cost and precision of climate model predictions exists.
Climate models were originally developed to help understand how the climate system works and so a hierarchy of climate models has evolved to answer different questions. These climate models can have very different characteristics, fit for different purposes.
The user needs to be aware of the strengths and weaknesses of each type of model to harness their capability, or to appropriately use data generated by these models.
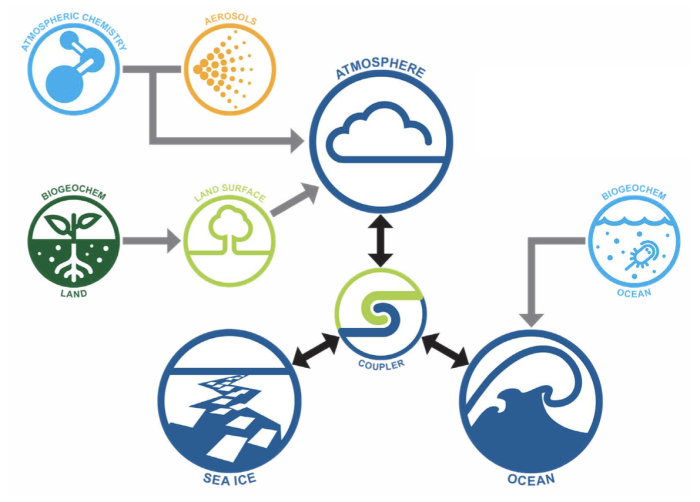
Figure 2: Australia has its own climate model – the Australian Community Climate and Earth System Simulator (ACCESS) https://www.access-nri.org.au/models/
What do climate models represent?
There are many types of climate models, and they vary widely in complexity. That is, the list of processes and the amount of detail each process is represented by, and the grid-spacing of the simulation. This affects their suitability to answer certain questions.
A contemporary physical climate model has around 50-100 layers in the ocean and in the atmosphere and each grid box is 50 – 200 km square in the horizontal.
There are models with much higher resolution than this (much higher spatial detail is represented), but global climate simulations for climate projections rarely use resolutions finer than ~50 km due to computational cost.
Climate models (Figure 2) will always contain approximations of some key processes, particularly key processes that act on scales smaller than a climate model grid-box, or where the process is not fully understood.
For example, a 100-km grid box model excludes thunderstorms, which are of the scale of a few kilometres, and are re-introduced into the climate model by simpler statistical approximations, called parametrisations.
However, even a 1-km grid box model, that now does include thunderstorms explicitly, will not contain the small cumulus clouds that are precursors to thunderstorms.
Similarly, soils and vegetation vary a great deal over a 100-km box and affect how the land evaporates, or heats the atmosphere, or how vegetation and soils help absorb carbon from the atmosphere. These are processes that have to be represented by their statistical properties or parameterisations.
Decision-makers should be aware that anticipating detailed information such as where to build a dam in a particular location, what crops to grow in in a particular region or which investment strategy a business with assets across the globe might employ requires an awareness and understanding of how and what climate models are telling us.
Types of climate models
Over the course of climate model development, two broad types of climate models have emerged as the most comprehensive and relevant to understanding how our climate has changed, or might change in the future, in the context of regional to global scales. They go by several names, the most common being General Circulation Models or Physical Climate Models, and a close cousin Earth System Models. Regional Climate Models are close cousins of their global counterparts.
There is no one-fits-all classification of climate models and there are examples that do not fit neatly into any classification. For example, the boundaries between Earth System and Physical Climate Models sometimes reflect some fundamental differences, and sometimes reflect a nuanced view of a process.
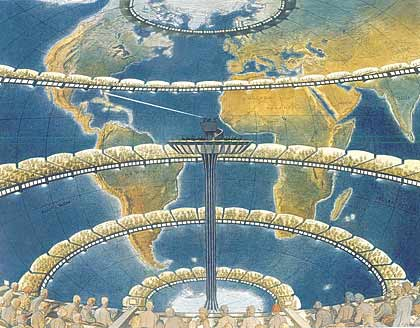
Computers use maths, data and computer instructions to create real world representations. Researchers identify rules about features and express those rules with maths. A complex system like the climate means solving thousands of equations taking months or years by hand. And what if you make a mistake?
Meteorologist Lewis Fry Richardson, creator of the first dynamic model for weather prediction, described a fantasy weather forecast “factory” of sixty-four thousand human computers working in “a large hall like a theatre,” calculating the world’s weather forecasts from meteorological data supplied by weather balloons spaced two hundred kilometres apart around the globe.
Currently the Gadi supercomputer hosted at the Australian National University, by contrast, solves 9 quadrillion operations per second.
Approximations in models
The equations used in climate models must be solved on a computer using approximations to represent some processes – L.F. Richardson’s vision of 64,000 humans solving these equations in a large hall is not really feasible. Some models only include processes which are relevant at a particular scale. For example, ground water, permafrost or dust production might not be relevant to global average climate change but would be more significant in some specific locations. Aerosol particles in the atmosphere can be represented by prescribing fixed or time-varying regional patterns of their occurrence or fully simulated with the chemical reactions involved and their interaction with cloud particles.
The choice is determined in part by computational constraints but also the nature of the problem being examined with the climate model.
Some processes are not well enough understood to be included in climate models, such as detailed processes like frost heave (upwards swelling of soil during freezing conditions) or hydraulic cavitation in vegetation but these are unlikely to be material to the Earth’s climate. However, they may be very important locally or regionally for assessing the impact of climate change.
It is not easy to unpick what processes a specific climate model includes or omits, at what level of detail or how well a process is simulated just from the data provided to users. The unwary should be very cautious and seek advice from those with detailed knowledge who often have long-term collaboration with these climate modelling groups.
How does the CMIP project feed into the IPCC?
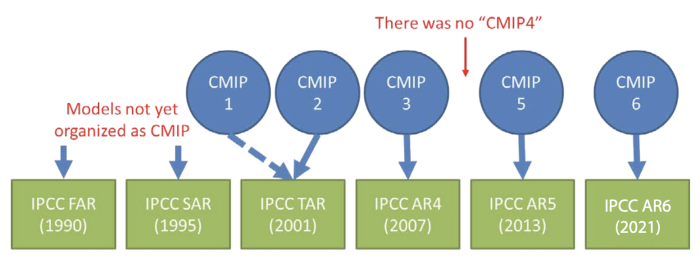
The Coupled Model Intercomparison Project (CMIP), is conducted by the World Climate Research Program (WCRP) and seeks to better understand the past, present, and future of our climate. CMIP began in 1995 with the goal of conducting a series of climate simulations with different climate models that all use the same agreed change in atmospheric carbon dioxide.
This allowed evaluation and comparison of climate models. CMIP is now in its 6th phase, CMIP6 (Figure 3).
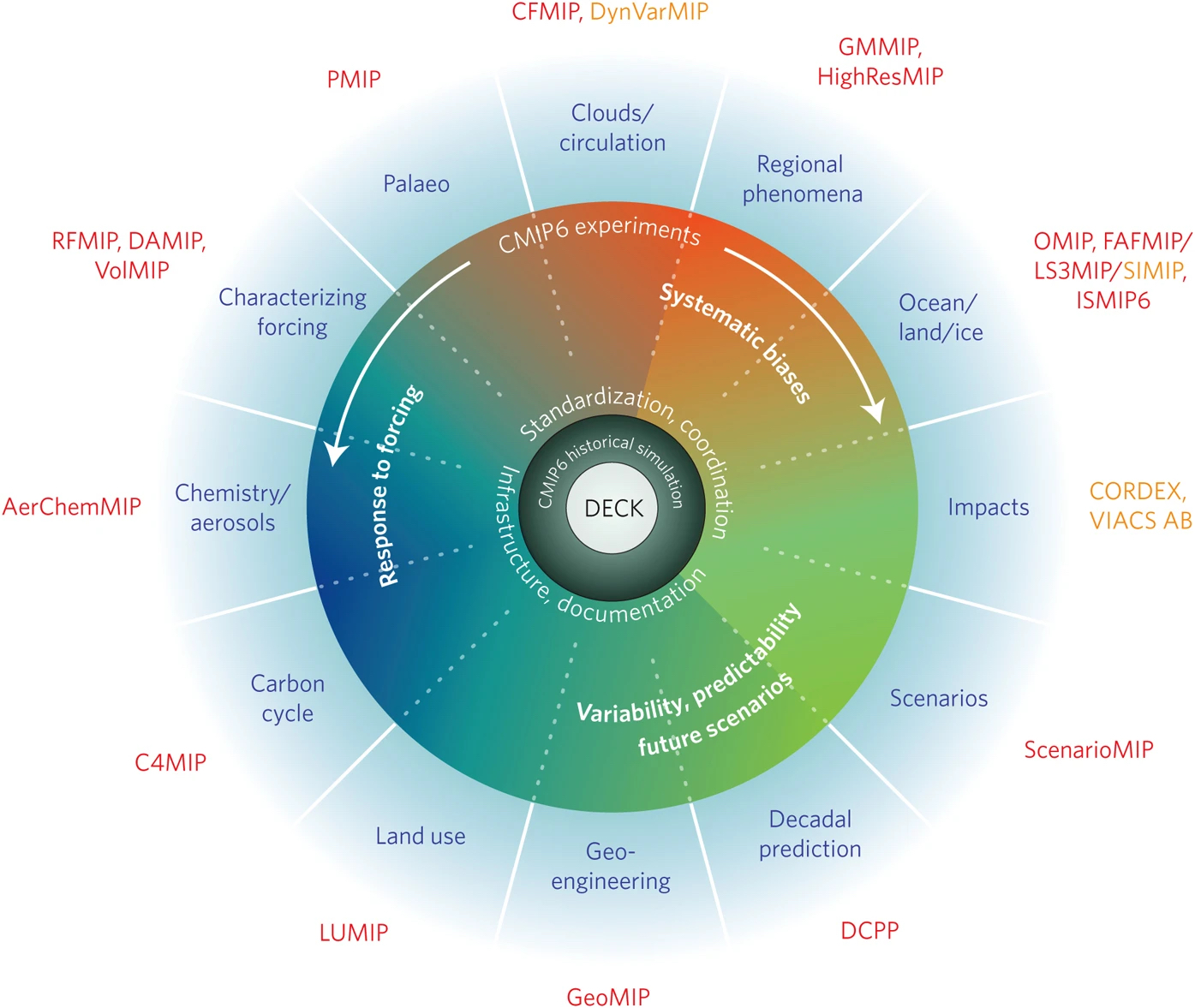
An important goal of CMIP is to make the multi-model output (Figure 4) publicly available to climate scientists in a standardised format.
CMIP6 data sets are very large using petabytes of storage (the storage >75,000 standard laptops) requiring specialised storage solutions coupled with high performance computers.
Modelling groups that carry out an agreed set of simulations can submit their data to CMIP6 to help grow the data set. The multiple models that form the CMIP6 data set are known as an “ensemble”. CMIP is an “ensemble of opportunity” since anyone can submit to CMIP using models of different standards or models that include varying representation of processes. A varied set of approaches helps climate scientists to study the intricate effect that different model choices have on the results.
For decision making, it is important to understand how each model works, the simplifications employed, the quality of the simulations, as well as the dependencies and interdependencies between the various models.
Although CMIP and the Intergovernmental Panel on Climate Change (IPCC) are independent, the CMIP models provide a source of information for the IPCC process, including a large suite of climate simulations using different scenarios of future human emissions of greenhouse gases.
The CORDEX project
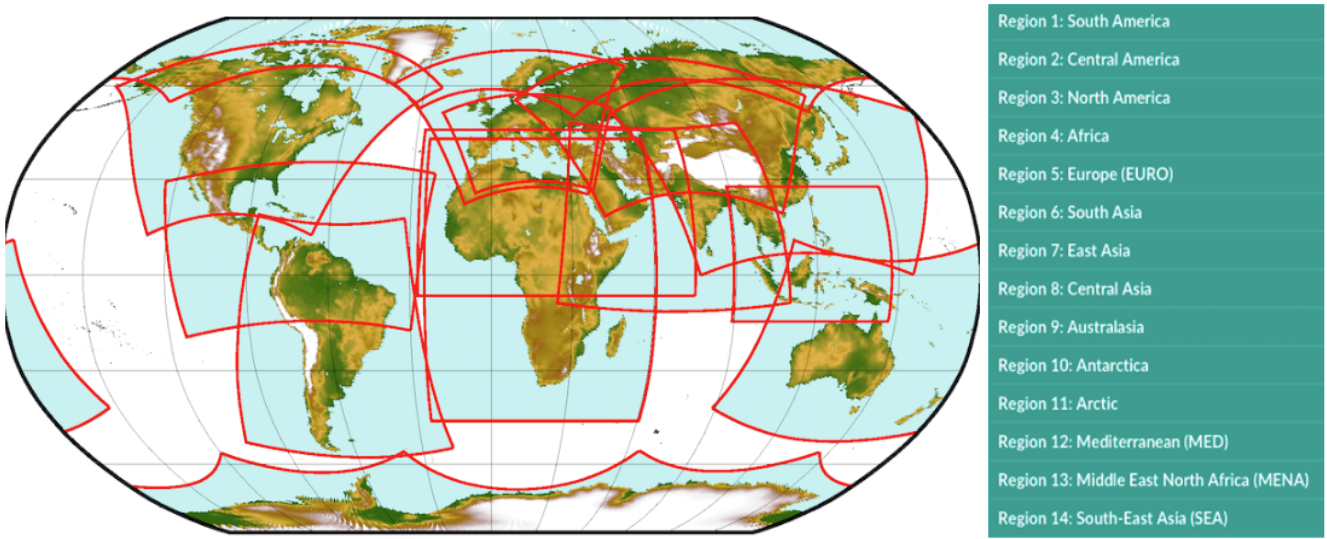
Figure 5: Boundaries of the fourteen official CORDEX domains. Image: https://cordex.org/data-access/regional-climate-change-simulations-for-cordex-domains/
As part of the WCRP, a project called the Coordinated Regional Climate Downscaling Experiment (CORDEX) (Figure 5) was designed as a strategy to develop regional downscaling methods and as an opportunity to engage a broader community of climate scientists in its activities. CORDEX uses Regional Climate Models.
The global Physical Climate Models and Earth System Models tend to use a relatively coarse grid owing to the computational cost of running these models. As a strategy to overcome this coarse grid, CORDEX coordinates “dynamical downscaling” activities over about 14 regions worldwide. The goal is to provide information on finer scales, with the most recent CMIP6 simulations downscaled to 25km and sometimes 12.5 km.
Dynamical downscaling allows the simulation of processes at 25 km and 12.5 km which is important for features of the atmosphere and ocean such as mountains, valleys, and coastlines which can be modelled more realistically. The 12.5 km simulations can resolve meteorological features that might intensify some extremes including the nature of some weather patterns.
Using models for future climate
Dynamical downscaling for future climate requires either a Physical Climate Model or an Earth System Model to first simulate the future climate for the whole globe.
This is used as input to regional models at the boundary of the region of interest. Data from the global climate models are provided at the boundary of a region every 3 or 6 hours as the regional simulation is conducted. Some large-scale assumptions in these boundary conditions (for example, the location of major weather features such as the storm tracks or the simulation of the ENSO phenomenon) can mean that the regional simulations are affected by assumptions contained in the global models.
Downscaling is dependent on how well the global models capture the large-scale climate because the regional models absorb information from the global models across a boundary at the edge of the area of interest. Running regional models at higher resolution is also computationally expensive. As a result, only a small subset of the CMIP models can usually be used to provide the information required at the boundary – unfortunately there is not enough supercomputing available to downscale every CMIP6 model. This limitation is being examined using machine learning.
Regional models are much higher resolution than global models, but this does not make them necessarily better for exploring future climate change. As with the CMIP6 data archive, users of CORDEX data need to understand the nature of the data, how it was generated and both its strengths and weaknesses. As Plato knew: “A good decision is based on knowledge and not on numbers”.
Summary
Climate models, based on fundamental laws of physics coupled with our detailed understanding of a range of important processes in the ocean, atmosphere and land, have led to many major advances in our understanding of our climate system. In addition, climate models have proven remarkably accurate in predicting the observed warming trend.
Groups around the world use climate models to simulate the future of our climate and these simulations are a rich data set for many important applications. However, the words “climate model” hides a vast array of complexity, differences, strengths and weaknesses for specific applications. Users of climate model data need to be cautious in using climate model data – and discussions with those building and running the models is a good way to rapidly build understanding.
The ongoing development of the software system called the Australian Community Climate and Earth System Simulator (ACCESS) (Figure 2) is now supported by the Federal Government via the National Collaborative Research Infrastructure Strategy (NCRIS). The ongoing development of the science incorporated in ACCESS reflects efforts by CSIRO, the Bureau of Meteorology, the ARC Centre of Excellence for Climate Extremes, and from 2024 the ARC Centre of Excellence for the Weather of the 21st Century.
Briefing note created by Professor Andy Pitman, Professor Christian Jakob and Professor Andy Hogg.
Reference list and author bios available in the PDF version below.
Climate model stakeholder
How do you choose the right climate model?
What does climate model resolution mean?